Perovskite LED
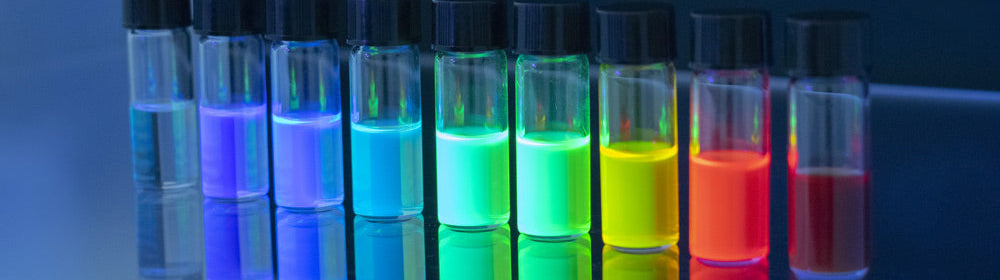
A perovskite LED (light emitting diode), often abbreviated to PeLED, uses a perovskite material layer to emit light. PeLEDs have many benefits over the common OLED (organic light emitting diodes) and QLED (quantum dot light emitting diodes) alternatives.
PeLEDs are predicted to be employed in a variety of applications. These include:
- Touch sensitive device displays
- Barcode scanners
- Night vision displays
- Medical monitoring such as photoplethysmography
Why Use Perovskites in LEDs?
Perovskites are a class of materials with the general structure ABX3, where A and B are cations and X an anion, typically a halide.
Perovskites have a wide range of beneficial properties.
- The solution processability of perovskites allows them to be deposited on a wide range of substrates, including flexible ones. This versatility broadens the scope of devices that can incorporate perovskite LEDS, including flexible displays like wearable electronics.
- Fabrication from solution-based methods makes PeLEDs a cheap alternative, as the deposition techniques such as spin coating or printing are less energy intensive and easily scalable.
- Perovskites also offer high efficiencies, in terms of both power conversion efficiencies (PCE) and external quantum efficiencies (EQE), for both solar conversion and light emission.
- Furthermore, due to the possible combinations of A, B and X, perovskites have a tuneable bandgap. As the bandgap directly relates to the wavelength of light emitted, this tuneability allows for different coloured LEDs.
These properties mean that Perovskite LEDs could present a cheaper alternative to current LED systems.
Advantages of Perovskite LEDs
There are multiple factors that make perovskite materials an exciting choice for use in LEDs. The most important of these are listed here.
- High Quantum Efficiencies
EQEs of of 28.1% have been achieved with a green emitting quasi-2D PeLED . The internal quantum efficiencies (IQE) of PeLEDS are rapidly approaching 100% and PLQEs as high as 99.8% have been recorded for 2D perovskite LEDs using CsPbI3 .
- Wide Range of Colours
The tunability of the perovskite bandgap means a wide range of colours can be emitted by PeLEDs. Bandgap engineering means all colours in the visible spectrum are accessible. A common approach to tuning the bandgap is incorporating mixed halides at the X site. For example, photoluminescence of a CsPbI3 perovskite quantum dot LED emits at about 680 nm, perceived as a deep red, whereas CsPbBr3 emits at 520 nm as green light. Mixing bromine and iodine at the X site in different ratios allows emission across the visible range, from purple to red. These results have been reproduced with methyl ammonium (CH3NH3) occupying the perovskite’s A site. Similarly, halide mixing in CH3NH3Pb(ClxBryIz)3 resulted in emission from 390 to 790 nm .
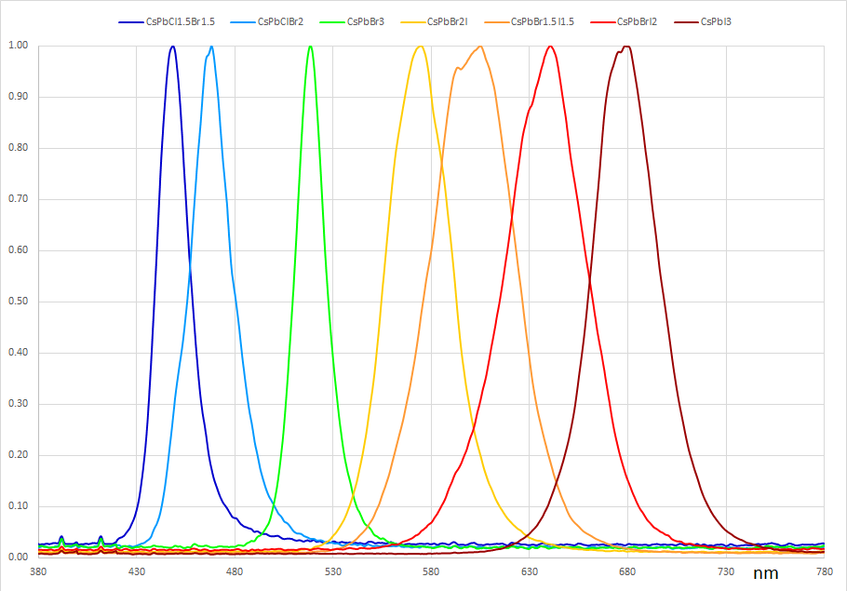
- Brightness
Many properties of perovskite make them ideal for photon emission. These include:
- high carrier mobility
- low non-radiative recombination rates
- low exciton binding energy
- high defect tolerance.
With these, PeLEDs have been proved to be up to 1000 times brighter than their OLED counterparts with irradiance values of over 40 W cm-2 .
How do perovskite LEDs work?
The mechanisms by which an LED operates opposes that of a photovoltaic cell. Rather than using light to produce energy, like in photovoltaics, LEDs use electrical energy to produce light. LEDs are comprised of a semiconductor emitter layer, in this case perovskite. Electrons are injected into the conduction band via an external circuit. They then recombine to emit light. The bandgap required for use in LEDs is between 1.7 to 3.2 eV, depending on the colour desired.
LEDs rely on the principle of electroluminescence (EL). EL involves the radiative recombination of electron hole pairs. This electron-hole pair is created after experiencing an injected electric current. Upon recombination a photon is emitted, with a wavelength corresponding to the bandgap, and this is the coloured LED seen.
Light from LEDs can also be generated from the recombination of excitons. These are a type of electron-hole pair, in which the electron and hole are tightly bound by Coulombic forces. In order for this exciton to recombine, it must overcome the excitonic binding energy. Therefore, the photon emitted is of a lower energy than the bandgap of the material.
Perovskite LED Structure
In perovskite LEDs, the emissive perovskite layer is sandwiched between two electrodes. Additional layers are also used for efficient PeLEDs, such as:
- Injection layers are used for both holes and electrons, they efficiently direct carriers to the perovskite layer.
- Electron and hole transport layers are respectively selective, therefore enhance the carrier mobility and prevent recombination outside the emissive layer.
- Buffer layers block shunting pathways, therefore, also contribute to an increased efficiency.
- Blocking layers prevent charge carriers traveling to the wrong electrodes.
Perovskite LED Fabrication
Depositing perovskite for LEDs uses the same method as for solar cells. Initially metal salts are dissolved into a solvent to form the perovskite precursor, or perovskite ink as it is often called. The ink is then deposited onto the chosen substrate, typically this is done by spin coating or slot-die coating. To encourage crystal growth, the films are annealed. Annealing conditions are dependent upon the A, B and X combination of the perovskite.
By using a solution-based approach, the fabrication of perovskite LEDs is simple and cheap. Similarly, perovskites can be easily deposited onto various substrates and thus a range of device types.
Challenges with Perovskite LEDs
- Exciton effects
Due to the low exciton binding energy of perovskites, excitons can readily dissociate into free carriers. These free carriers can then recombine non-radiatively. No photon is emitted, which reduces the PLQE and increases the leakage current of the device. PLQE can be used to measure this.
- Stability
A substantial weakness of PeLEDs compared to OLED and QLEDs is their operational lifetime. Perovskites experience ion migration and lattice strains. When perovskites are used in LEDs and subjected to electrical stress, the material degrades faster, and this can lead to non-emissive areas (dark spots). Current research is being done to overcome these issues. For example, pimelic acid has been used as an additive to prohibit interfacial reactions in PeLEDs which have achieved an operational half-life of 682 hours under a radiance of 17 W sr−1 m−2 . Under 1000 cd m-2, the half-lives of blue, green and red perovskite LEDs are recorded as 0.4, 11 and 112 hours respectively .
- Blue LED efficiencies
Blue PeLEDs perform considerably worse compared to green and red perovskite LEDs. Blue light has the shortest wavelength of these, less than 475 nm, and therefore requires the largest bandgap (over 2.6 eV) to emit photons. To achieve such a wide bandgap, a mixture of halides is employed at the X site of perovskite, often chlorine and bromine. However, under bias the halides segregate into separate domains causing inconsistent photon emissions and a decreased EQE. One example of segregation passivation used a 3D/2D device structure and an yttrium chloride additive, this resulted in a blue PeLED with a PLQY of 49.7% . Although a considerable improvement, this is not yet competitive with green or red perovskite LED results.
Perovskite Materials
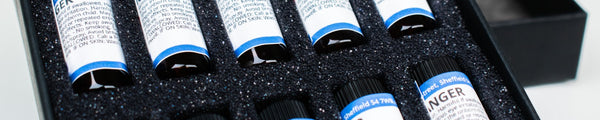
Learn More
References
- Perovskite Light-Emitting Diodes with EQE Exceeding 28% through..., Z. Liu et al., Advanced Materials (2021)
- Ultrastable and highly efficient green-emitting perovskite quantum dot..., T. Xuan et al., Nano Energy (2022)
- Emerging Self-Emissive Technologies for Flexible Displays, D. Zhang et al., Advanced Materials (2019)
- Electrically assisted amplified spontaneous emission in perovskite light-emitting..., K. Elkhouly et al., Nature Photonics (2024)
- Critical role of additive-induced molecular interaction on the..., C. Kuang et al., Joule (2021)
Further Reading
Perovskite light-emitting diodes. Fakharuddin, A. et al., Nature Electronics (2022)
Device Structures, and Applications of Flexible Perovskite Light-Emitting Diodes. Jang J. H. et al., Materials (2023)
Ultrawide Color Gamut Perovskite and CdSe/ZnS Quantum-Dots-Based White Light-Emitting Diode with High Luminous Efficiency. Lin, C.-H. et al., Nanomaterials (2019)
Towards micro-PeLED displays. Yang, X. et al., Nature Reviews (2023)
One-dimensional organic lead halide perovskites with efficient bluish white-light emission. Yuan Z. et al., Nature Communications (2017)