How Do Batteries Work: An Introduction to Batteries
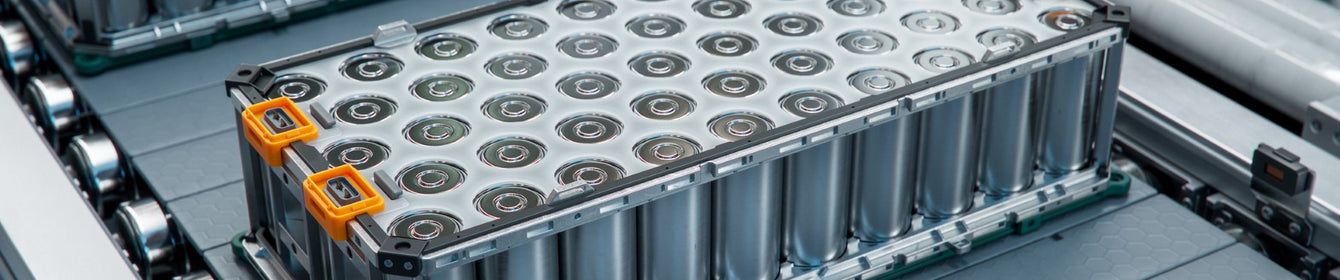
A battery is an energy storage device which provides an easily accessible supply of electrical energy. Batteries convert chemical potential energy, from redox reactions, into electrical energy.
Redox reactions include both reduction (where electrons are gained) and oxidation (where electrons are lost) reactions. These happen simultaneously as charge is transferred from one species to another.
Since the invention of the first battery in 1800, batteries have proven their versatility by powering everything from small consumer goods like toys to large vehicles. Over time, a wide range of battery materials with varying chemistries, such as lithium iron phosphate (LiFePO4) powder and lithium cobalt oxide, have been developed to suit different applications.
How Do Batteries Work?
A common example of stored electrical energy is when a plastic rod is rubbed with fur. The rod becomes negatively charged as the electrons are transferred from the fur. Batteries use a more efficient method to transfer charge: redox reactions. Batteries convert chemical potential energy, from the redox reactions, into electrical energy and consist of four key components:
Component |
Role |
---|---|
Electrode (cathode and anode) |
Enable output and input of electrical energy, these are where the redox reactions occur. |
Electrolyte |
Transfers ions and facilitates a controlled electron flow through the circuit. |
Separator |
Creates mechanical stability and prevents immediate discharge. |
Battery management system |
Maintains a safe voltage region to prevent overheating. |
Redox reactions involve the transfer of electrons from one species to another. Therefore, some species lose electrons (oxidation) and some species gain electrons (reduction). Whether the oxidation or reduction reaction takes places at the anode or cathode depends on whether the battery is charging or discharging, this also dictates the direction of ions between the electrodes. A typical set of redox reactions for a Li-ion (Li+) battery is shown below:
Full redox reaction: LiC6+ CoO2 ↔ C6 + LiCoO2
left to right is discharging (→), right to left is charging (←)
Storing Electricity
Batteries store electrical energy by converting it into chemical potential energy. This is defined as ‘charging’ the battery. An applied voltage drives ions stored in the cathode towards the anode via the electrolyte. When the ions reach the anode, they are positioned within the anode material’s layers. This process is called intercalation. At the same time, electrons move from the cathode to the anode through the circuit. The non-conductive nature of the electrolyte and the separator prevent the electrons travelling back towards the cathode, known as internal short circuiting. Therefore these electrons are held at the anode, ready for discharge.
The type of ion depends on the type of battery, for example lithium-ion batteries have cathode materials that supply lithium ions (Li+).
Releasing Electricity
Connecting the battery to a circuit causes the battery to release electricity, this is known as discharging. The circuit allows for a flow of electrons from the anode back towards the cathode without an applied voltage. This releases the stored electrical energy.
Types of Battery
Batteries have been under development since 1800 and therefore there is a wide range of variations. The different chemistry used in batteries each offer unique benefits and applications.
Primary and Secondary Batteries
Batteries can be organized into two main categories: primary and secondary. Primary batteries are single use, while secondary batteries are rechargeable. Both can be further divided into subcategories depending on the battery materials used.
Primary batteries are typically assembled charged and the lifespan depends on the amount of chemically reactive material used. Secondary batteries still have a limited lifespan, known as the lifecycle. The lifecycle of a secondary battery is based on how many times the battery can be fully recharged until the capacity of the battery drops to below 80% of the original capacity. The rechargeability of a secondary battery stem from the reversibility of its redox reactions.
While primary and secondary batteries have their differences, most consist of the same components.
Battery Type | Usage | Lifespan | Examples | Applications |
---|---|---|---|---|
Primary |
Single use |
Finite, depends on amount of chemically reactive material |
Alkaline |
Consumer electronics, such as smoke detectors, flashlights and toys. |
Secondary | Rechargeable |
Limited, known as the lifecycle. This is how many charge cycles can occur before capacity drops below 80% |
Lithium-ion Lead-acid Nickel-cadmium |
Consumer goods and electric vehicles Car batteries Emergency power source |
Battery Applications
Battery applications depend on a range of characteristics, including electrical storage capacity, high power, and lifespan. Different batteries are known by their chemistries and research is highly focused on optimizing and discovering further applications.
Lithium-Ion
Lithium-ion (Li-ion) batteries are the most common type of secondary battery. Most often these are used in electrical goods and electrical vehicles (EV). Lithium-ion batteries offer a high energy density, long cycle life, and are lightweight.
Alkaline
Alkaline batteries are another common type of primary batteries and are often used to power consumer electronics such as smoke detectors, flashlights and toys. Alkaline batteries are reliable and have long shelf lives. As well as their inability to recharge and lower power outputs, they are also commonly made of toxic materials and so leakages can be dangerous if mishandled.
Lead-Acid
Commonly used in the automotive industry, lead-acid batteries are reliable secondary batteries that can provide large currents. The ability to offer a high and sudden charge is required for starting a car engine, especially in colder climates.
Nickel-Cadmium
Nickel-cadmium (Ni-Cd) were once popular; however, these secondary batteries have been replaced by safer options due to environmental concerns. While they are not as common, Ni-Cd batteries are still sometimes used as an emergency power source as they deliver reliable power over a long lifecycle.
Measuring the performance of a battery
Discharge rate
Typically referred to as C, the discharge rate affects how quickly charge flows through a circuit. A battery with a discharge rate of 1C can discharge its capacity in 1 hour. The higher the C value the faster the battery can discharge. If a battery holds 2000 mAh but can discharge with a current of 1000mA, then the battery has a C rate of 2C.
C is determined by the ion mobility and overall capacity. If the lithium ions in a lithium-ion battery can move through the electrolyte faster, the electrons at the anode can discharge through a circuit faster. Similarly, during charging, the lithium ions can be stored in the anode faster.
Charge capacity
The specific capacity of a battery is defined as how much charge can be stored per unit mass: mAh/g. The theoretical capacity can be calculated by:
Where Q is the specific charge, n is the number of electrons transferred per mole of reaction, F is Faraday's constant, and Mr is the molecular mass.
The experimental capacities are far inferior to the theoretically calculated. This is due to imperfections and defects in the purity of the material. For example, LiCoO2 has a theoretical capacity of 274 mAh/g but only an experimental capacity of 165 mAh/g.
Energy density
Energy density is the amount of energy a battery can store per unit mass and is measured in watt-hours per kilogram (Wh kg-1). Higher energy densities mean more energy can be stored in a smaller energy. More stored energy increases the lifetime of the battery and means it does not need to be replaced as frequently. Moreover, more energy in a smaller area means more compact and lightweight batteries can be fabricated. This is particularly important for the usability of consumer goods such as smartphones and laptops as well as in electric vehicles.
Lifetime
The lifetime of a battery is one of the most important properties to the consumer. A battery ‘s lifetime is defined as the number of times a battery can be fully recharged whilst maintaining at least 80% of its original capacity. Often this is stated as a timeframe, in hours, instead of a number of cycles. Not only do longer lifetimes increase the relative efficiency of a battery, but they also increase user convenience. Long lifetimes mean less replacements and less waste, therefore increasing the sustainability as less energy is required to both produce and recycle the battery.
Battery Research
A vast amount of research is ongoing to determine the best chemistry to boost each aspect of a battery. Specifically, there is a large focus on lithium-ion batteries due to their use in EVs. While Li-ion batteries are the dominant rechargeable batteries, there is potential for new battery developments. For example sodium-ion batteries (Na-ion), there is a natural abundance of sodium, making it easier and cheaper to obtain than lithium. Similarly, graphene batteries are advancing due to their high energy density and non-flammable makeup.
Explore the range of high-purity cathode materials designed for high-capacity, high-voltage batteries to maximize energy density.
Learn More
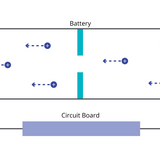
Batteries can be recharged by plugging them into a power source, which initiates electrochemical reactions causing charged species within the battery to return to the anode. Restoring the battery’s potential energy.
Read more...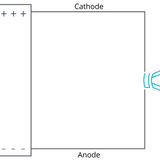
Defining a cathode and anode as positive and negative, or as the source and sink of a current, depends on your definition of current itself. Current can describe the flow of positive or negative charge.
Read more...